On the Path to Accessible Cell Therapies: Overcoming Current Challenges
By Dr. John Harrington, Executive Vice President and Chief Scientific Officer at Athersys
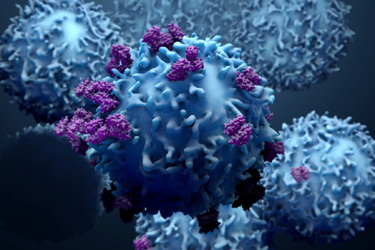
The capabilities of the biotech industry are stretching further than previously thought possible. Thanks to research, manufacturing capabilities, and other forms of innovation, cell therapies that were once an aspiration for the distant future are now a reality.
The promise of cell therapies lies in the fact that they are essentially living drugs working in conjunction with a patient’s body. Cell therapies may address multiple indications and may promote the production or inhibition of target proteins and enzymes. Cell therapies can also be designed to enhance the growth of healthy cells, limit the replication of abnormal diseased cells, or exert other pharmacological effects on the body. Thus, a wide range of acute and chronic conditions can benefit from the capacity of cell therapies to operate in the body in a way that is tailored directly to the patient, providing the desired effect at the intended site of action.
Many of the challenges therapeutic developers face with cell therapies are related to the fact that cells are alive and require specific conditions to maintain their viability, potency, and overall efficacy in a therapeutic setting. Every part of the supply chain must integrate specific requirements to keep cells alive, from manufacturing to shipping and storage, to thawing and administering the product. Thus, control must be maintained throughout all aspects of the supply chain, beginning with manufacturing all the way through to the administration of the product to the patient.
Administration of cell therapies addressing certain clinical indications, such as oncology, can often be scheduled weeks or months in advance due to the chronic nature of the disease. As a result, cell therapies for these chronic indications can be manufactured and delivered to the clinical site on an as-needed basis. Acute clinical indications, on the other hand, require that the product be manufactured and stored at the clinical site in advance since, in most instances, the product must be administered to the patient within hours or days of injury or disease onset. In addition, allogeneic cell therapies often require ultra-low temperature storage, necessitating the need for clinical sites to utilize specialized freezer units on site. Accordingly, cell therapy manufacturing can be significantly more complex when developing allogeneic cell therapies designed to treat acute conditions. The short-term, unexpected timing of health conditions such as stroke and acute respiratory distress syndrome (ARDS) can place pressure on the drug developers’ supply chain and appropriate steps must be taken to ensure the product is available when and where it is needed.
Manufacturing
Before any drug can be used in a clinical setting, it must first be manufactured under stringent and regulated conditions. Regardless of whether it is a chemical compound, an antibody, or a cell therapy, it must be produced in sufficient quantities to serve clinical and commercial needs. While small molecule compounds are synthesized and antibodies are produced from recombinant cells, cell therapies are themselves living organisms. As a result, the entire manufacturing process, from upstream cell expansion to downstream purification, formulation, vialing, and freezing must be capable of maintaining cell viability.
Allogeneic cell therapies are sourced from a human donor and must then be isolated and expanded to generate billions or trillions of cells. Following the expansion of the cells, hundreds to thousands of liters of cell product must be concentrated, purified, formulated, vialed, inspected, and frozen in a matter of a few hours to maintain cell viability. In addition to the short processing times, all manufacturing steps must be carried out using gentle, low shear force processes to prevent cell lysis from occurring. Finally, as a further constraint, the entire cell therapy manufacturing process must be carried out aseptically, as terminal sterilization of the product is not possible.
Testing
During manufacturing, cell therapies undergo extensive testing to ensure the product is sterile, potent, and meets quality and regulatory requirements under current Good Manufacturing Practice (cGMP) standards. This testing occurs throughout the manufacturing process and involves in-process and final release testing. In-process testing involves quality assessments that are carried out in “real-time” during a manufacturing step prior to advancement to the next step in the manufacturing process. One of the significant challenges associated with in-process testing for cell therapy products is that the product cannot be held for long periods of time while testing is being performed. As a result, some in-process tests, such as sterility, are not possible due to their long test periods while others must be performed on an expedited basis. Since allogeneic cell therapies are typically stored frozen following manufacturing, the more extensive product release testing must be carried out after the product has completed the manufacturing process.
Shipping and Storage
Storage and distribution of cell therapy products pose additional challenges. Cells lose both their viability and potency over a short period of time under inadequate conditions. Whereas antibodies and small molecule drugs are relatively stable, often at ambient temperature, cell therapy products must be maintained in the frozen state at ultra-low temperatures below the glass transition temperature, generally below minus 120 degrees Celsius. To accomplish this, validated shipping containers capable of maintaining these low temperatures for up to 1 week are utilized to facilitate the transfer of product from the manufacturing site to clinical sites around the world. Of course, ultra-low temperature freezers are required prior to shipment and at the clinical site following shipment for storage prior to use, and monitoring of temperature is necessary to ensure that temperature excursions have not occurred.
In addition to temperature control, cell therapies must also be treated with extra care during transit to avoid the risk of contamination or cell death. Of particular note, it is critical that measures be put in place to manage security inspections during shipment, such as X-ray interrogation of shipping containers, which could impact product performance for cell therapeutics. Companies can reduce the risks associated with many of these issues by labeling shipments of doses appropriately and using a dependable and regulatable shipment and distribution provider.
Once at the clinical sites, storing of cell therapies can be accomplished using freezers to store the necessary quantities of doses at ultra-low temperatures. Ideally, ultra-low freezers should be in the same building or complex where patients receive the treatment. Since most pharmacies and clinical sites are not accustomed to accommodating products stored at ultra-low temperatures, a certain amount of training and capital investment will be necessary at clinical sites as more cell therapies are introduced to the market.
Dose Preparation and Administration
Cell therapy products can be administered at a range of clinical sites, including treatment centers, specialty care centers, and hospitals. Unlike other types of drugs, such as antibodies and small molecules that often require minimal dose preparation, cell therapies typically involve a thawing and administration process that must be executed within a matter of hours by a pharmacist or clinician at a clinical care facility. Since the thawing process is critical to maintaining cell viability and potency, detailed procedures will be necessary for ensuring the integrity of the product. Ideally, automated thawing devices should be employed by the clinical sites to address these issues. In addition, procedures to ensure administration of the product to the patient within a short period of time will also be necessary.
Cell therapies are a new and promising therapeutic class within the biotech industry. An effective manufacturing process and supply chain are necessary to ensure that cell therapy products are ready for use in the clinic in sufficient quantities, particularly for acute clinical conditions. Cell therapy developers are at the forefront of innovation in our industry and will continue to develop new solutions for manufacturing, distributing, storing, and administering cell therapies to the patients who need them most. This includes extensive networks of cold chain storage, temperature regulation and monitoring capacities, and communication across the supply chain. As the field evolves, hospitals and other clinical sites using cell therapies should incorporate best practices for storing and administering cell therapy doses, especially when the cell therapy is used in urgent, life-threatening cases of acute indications. Mastering the supply chain is critical to enable cell therapies to reach their full potential.
Dr. Harrington co-founded Athersys in 1995 and has served as our Chief Scientific Officer, Executive Vice President and Director since our founding. Dr. Harrington led the development of the RAGE® technology, as well as its application for gene discovery, drug discovery and commercial protein production applications. He is a listed inventor on over 20 issued or pending United States patents, has authored numerous scientific publications, and has received numerous awards for his work, including being named one of the top international young scientists by MIT Technology Review in 2002. Dr. Harrington has overseen the therapeutic product development programs at Athersys since their inception and is also focused on the clinical development and manufacturing of MultiStem®. During his career, he has also held positions at Amgen and Scripps Clinic. He received his B.A. in Biochemistry and Cell Biology from the University of California at San Diego and his Ph.D. in Cancer Biology from Stanford University School of Medicine.